How to Pick the Optimal Rocket Engine
From ion thrusters to staged combustion engines
Rocket engines come in much larger variations than car or airplane engines. But why is there so much variation and not more standardization? Why not use the same type of engine in different sizes depending on how big the rocket is?
The key driver of the great variation of rocket engine design is the conflicting tradeoff between power and efficiency. Very powerful engines tend to be inefficient, while very efficient engines tend to be weak. A powerful rocket engine has high thrust. Thrust is usually measured in Newtons.
1 Newton (N) is the force that causes an object of mass 1 kilogram (kg) to increase its velocity by 1 meter per second every second (m/s²).
As an example, gravity on earth exerts a force of 9.8 Newtons on an object of mass 1 kg. An object of 10 kg would experience a gravitational pull of 98 Newtons.
The measure of efficiency of a rocket engine is a little harder to grasp. When talking about cars, we will relate engine efficiency to kilometers per liter of fuel. To understand efficiency metrics for rocket engines, in contrast, it helps to contemplate how you would measure efficiency of a car engine if it was not installed in a car. In that case, you would look at how quickly the fuel tank would get drained while it outputs a certain amount of force. We can analyze a rocket engine in much the same way. We measure how quickly a rocket propellant tank is depleted in terms of mass flow (kg/s).
Furthermore, we obtain the rate of depletion by dividing the thrust of the engine by the exhaust velocity of the propellant. Allow me to clarify: A rocket engine burning hydrogen will emit very hot water vapor, which later cools into small water droplets. That is why you see a large white cloud forming behind a rocket as it ascends into the sky. The exhaust velocity of the engine is the velocity of these water molecules when they exit the rocket engine.
Isn't that kind of the same as the temperature of the exhaust? Not quite. Temperature is a measure of average kinetic energy of the particles within the substance you measure the temperature of. The kinetic energy of a particle, in contrast, is determined not just by its velocity but also its size. Thus, small atoms with the same kinetic energy as large atoms will move at a higher velocity.
In other words, assuming the temperature of the gases expelled from the rocket engine is some value T, then lighter particles will give better fuel efficiency than heavy particles. That helps explain why an engine using hydrogen fuel gets higher efficiency but worse thrust than a kerosene engine. Engines running on kerosene will produce carbon dioxide (CO₂) and water (H₂O) which is heavier than pure H₂O. Many kerosene engines try to improve efficiency by running fuel rich, causing incomplete combustion resulting in carbon monoxide (CO). CO is lighter than CO₂ and thus gives better fuel efficiency.
If you are keen to learn more about rocket fuel, then check out the article I wrote specifically to discuss that topic:
Ion Thrusters vs. Kerosene Engines
The tradeoff between fuel efficiency and power also exists for cars. You can see how cars with a lot of horsepower also tend to have poor gas milage. For rockets, these tradeoffs can be far more extreme. To launch from a planetary surface, you generally need exceptionally powerful engines and are willing to throw a lot of efficiency overboard. However, once in orbit, fuel efficiency matters a lot more. Spacecraft built to travel into deep space, such as the Dawn probe visiting the dwarf planet Ceres, require very efficient engines to travel that far. Dawn uses ion drives for propulsion. An ion drive is basically a particle accelerator turned into a rocket engine. The atoms in the propellant are ionized and then accelerated in an electrical field before they get released from the engine into space.
The Deep Space 1 spacecraft, powered by an ion thruster, changed velocity by 4.3 km/s while consuming less than 74 kg of xenon. Thus, with no more fuel than would fit into two large automobile gasoline tanks, the Deep Space 1 spacecraft increased its velocity with 15 480 kilometers per hour. That corresponds to 9619 miles per hour.
Such massive velocity increase is possible because Ion thrusters are ten times more propellant efficient than a normal kerosene or hydrogen rocket engine. That efficiency comes at a cost. Ion thrusters are very weak. Typically, they have a thrust of 25–250 millinewton (mN). A single Merlin 1D engine used on the Falcon 9 rocket has 845 kilonewton (kN) of thrust. In other words, a Merlin 1D engine is 4-40 million times more powerful than an ion engine.
Another interesting fact about ion engines is that they are essentially electrical engines. To create the electric field which accelerates xenon ions, electricity is needed. Electricity can be supplied with solar cells. A typical engine consumes 1–7 kW. That is less than 10 horsepower.
Nuclear Thermal Propulsion Systems
While ion engines are efficient, they are not strong enough to power a manned mission to Mars. Such a mission will require a lot more cargo, and we want higher thrust to arrive at Mars faster. One could use chemical rockets, but because they are so inefficient we don't have enough propellant to fire the engines for very long. That means you have to spend 7 months in space before you arrive.
A possible solution is a nuclear thermal propulsion system (NTP). NASA is currently working with DARPA to develop such as system: NASA, DARPA Will Test a Nuclear Engine for Future Mars Missions.
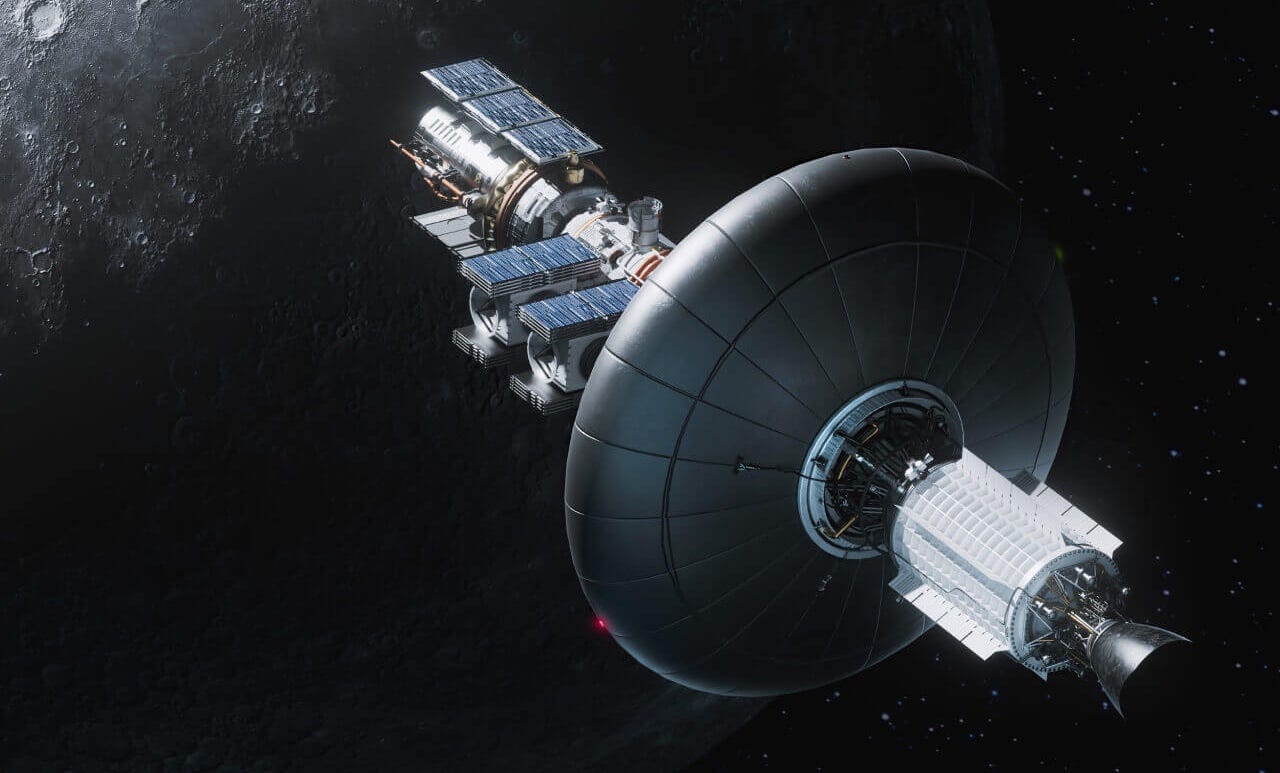
Remember, specific impulse is related to how fast particles are emitted from the rocket engine. A chemical reaction produces much less heat than a nuclear reaction. Thus, if you heat the propellant with a nuclear reactor instead of through a chemical reaction, you get much higher propellant efficiency. An NTP rocket gets more than twice the propellant efficiency of a hydrolox engine.
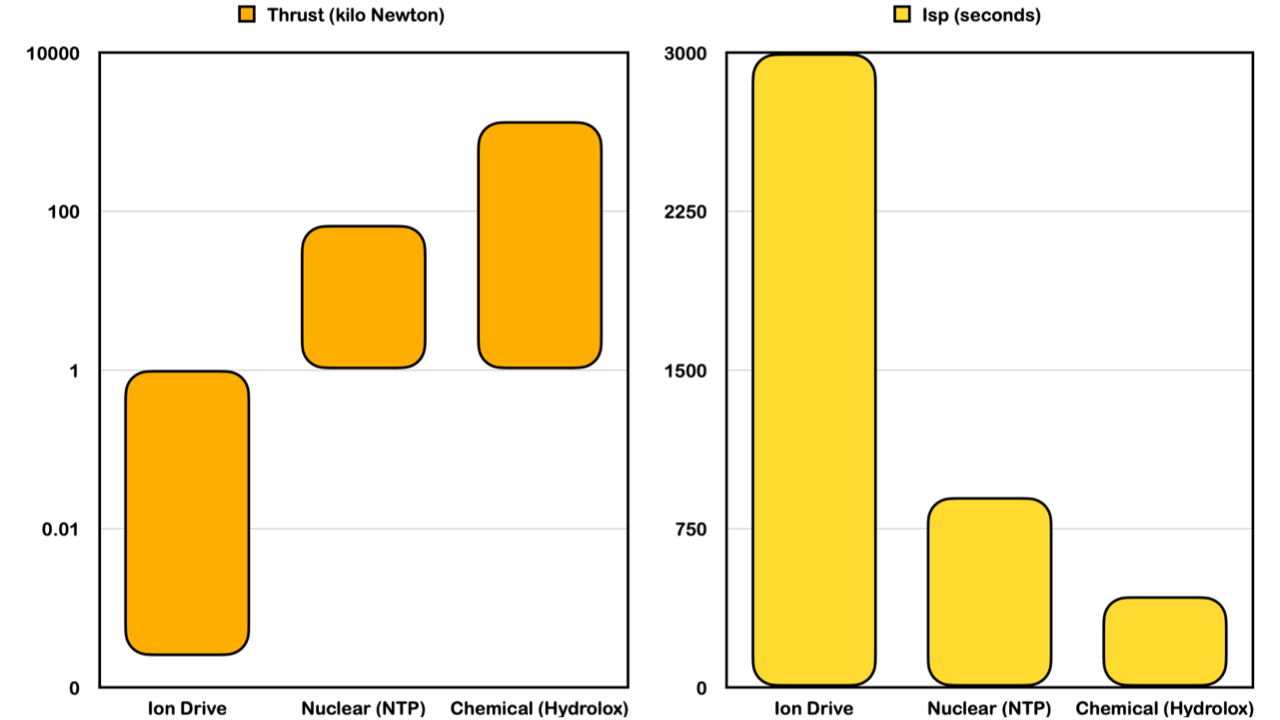
With that kind of propellant efficiency, you can cut the travel time to Mars down to 45 days, which offers numerous advantages:
Living weightless for 7 months will take a heavy toll on the crew, who will struggle to recover after landing on Mars.
Outer-space has a lot of radiation. The dosage received by the crew can be significantly reduced by shortening the trip.
One frequent source of confusion about nuclear propulsion which I like to point out is that propellant efficiency calculations only related to the propellant heated up by the reactor and not the uranium used in the reactor. You will likely spend the propellant long before you have exhausted the uranium fuel. The rocket reactor might be able to operate for several years before any refueling of the reactor core with Uranium will be needed.
The NTP engine I use in the chart above designed by USNC can be refueled with propellant 10 times.
Chemical Rocket Engines
Keep reading with a 7-day free trial
Subscribe to Erik Explores to keep reading this post and get 7 days of free access to the full post archives.